Introduction
Predicting possible side effects is a big part of the drug and medical devices development process. To meet the objective the proposed product goes through rigorous trials including benchtop, animal and human. Despite the underlying biology being similar, the sizeable biological differences that exist within the population and biological system change overtime and limits accuracy. In the end, animal and human experiments are also only experiments with errors and limited predictive power.
In medicine, biomechanics engineers capture and analyse movement patterns and translate them into computer models and simulations that are used to develop therapeutic interventions, medical devices, and medical software.
To cope up with the ever increasing modern biomedicine complexities modelling and simulation presented as a new method that can enable development of better and safer medicine and medical devices. Modeling and simulation is the application of computer models to analysis systems in a digital environment for better understanding.
Computational modelling and simulation tools are used to assess the condition of medical devices and biological systems under different conditions and predict the response using computers. The understanding of this prediction has helped reduce the dependency on traditional trial processes that are very expensive and time consuming.
In Silico Medicine - What is that?
In silico describes the evolution of medicine and is seen as the next evolutionary step: from in vivo, i.e. experiments in the human body, to in vitro, i.e. in the test lab, to in silico β experiments performed in silicon, i.e. on the computer.
The emergence of in silico medicine is the result of advances in medical informatics over the past twenty years. Scientists define βin silicoβ as the modeling, simulation and visualisation of biological and medical processes on a computer.
Digitisation is expanding the boundaries of medicine and medical technology. Computer modelling and simulation (CM&S) or in silico technologies combine computational tools with biology to perform complex analyses of life science applications in an intuitive, accurate, and reproducible manner.
There are a variety of potential applications for in silico models:
- In silico modelling is used in the pharmaceutical industry to calculate how the pharmacology and pharmacokinetics of a compound determined in laboratory and animal tests might act in humans. In addition, complex computer simulations of the physiology of humans and animals are used to make predictions about how a compound will act in humans, even before initial data from tests in humans are available. In silico models can thus be used to test entire populations virtually for drugs or various diseases without risk, and to improve the efficacy of drugs in a targeted manner.
- In silico models can also be used, for instance, for digital verification of implants or prostheses (example Simq VIT). By virtually applying physiological or standardized loads to a patient-specific situation, the performance and safety of an implant can be tested quickly and efficiently using objective criteria before it is implanted in the patient. The patient thus receives an implant that is perfectly tailored to his or her anatomy. In addition to the doctors and implant manufacturers, the patient also benefits from increased quality and safety.
- Another possibility is the use of in silico models for surgical planning. Here, medical software is used to optimize the operation based on simulation in order to achieve the best possible results for the patient while minimizing risks (example Simq RPE).
Application of CAE in Bioengineering
As geometric "CAD" planning slowly becomes state of the art in medicine, CAE is a logical next step. Computer aided engineering (CAE) is an overarching term used to represent different types of computer application for digital modelling of different engineering problems.
Based on the working computational and mathematical principle, CAE can be classified in different types. Based on its applicability it is mainly defined as the Finite Element Method (FEM) that is mostly used for structural studies (it can also be used for fluid mechanics applications) and Computational Fluid Dynamics (CFD) which is better suited for fluid flow problems.

Finite Element Analysis (FEA)
FEA has been used to study complex structural problems in humans for a long persiod of time in Biomechanical Reasarch. The biological systems such as bones, tissues, and implants have been studied using FEA to predict system response under different medical conditions.
Impact analysis FEA has been used to predict injuries in different scenarios and the outcome can then be used to design safety devices to prevent injuries. Even further initiatives apply FEA to model full human body parts that can ultimately be used to study stress, strain and kinematics in the human body.
Impact analysis FEA has been used to predict injuries in different scenarios and the outcome can then be used to design safety devices to prevent injuries. Even further initiatives apply FEA to model full human body parts that can ultimately be used to study pressure shocks in the human body.

FEA has also been used to investigate biomechanical performance of implants designed to have oversight over the effect of load on it and the surrounding bone. When it comes to prosthetics, FEA has a potential to model the stress and strain distribution in lower-limb prosthetic design. This is very difficult to measure in experimental setup. Its application has a potential to offer insight into soft tissue load distribution that can be input for design and material optimisation. The virtual testing of this product can significantly help to deliver high performance products cost effectively.
Computational Fluid Dynamics (CFD)
CFD has been used to model and simulate blood flow through the heart, arteries and other organs. The most famous example of CFD in the sector is the heart flow analysis.
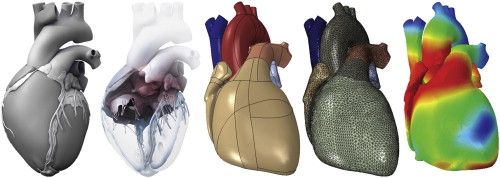
The application of CFD enables the first non-invasive test where medical professionals have the ability to study the effect of narrowing and blockage of arteries on the blood flow. The technology has facilitated rapid, cost effective and low risk prototype CFD simulation is transforming the cardiovascular studies. Researchers also used to optimise kidney dialysis devices.
Challenges & Benefits of Simulation in Biomedical Engineering
The integration of modelling and simulation techniques in biomedical engineering has ushered in a new era of innovation and efficiency in drug and medical device development. However, this approach comes with its own set of challenges and benefits that have significant implications for the industry.
Challenges
- Biological Variability: One of the major challenges in biomedical simulation is accounting for the wide variability in biological systems among individuals. While computational models strive to represent the average behaviour, individual differences can lead to variations in response. This variability can limit the accuracy of predictions, especially when attempting to generalise results to a diverse population.
- Model Validation: Validating computational models in biomedical engineering is a complex task. Ensuring that these models accurately represent real-world biological processes and responses requires extensive experimental data and iterative refinement. This validation process can be time-consuming and resource-intensive.
- Data Quality and Quantity: High-quality, comprehensive data is essential for building accurate computational models. Gathering, managing, and integrating diverse biological data sources can be challenging. Moreover, the quantity of data needed for sophisticated simulations can be substantial, necessitating advanced data acquisition and storage solutions.
- Algorithm Complexity: Developing accurate simulation algorithms for complex biological systems is a formidable challenge. The intricacies of cellular and physiological processes require sophisticated mathematical models and algorithms, often demanding significant computational resources.
- Ethical Considerations: Simulating biological systems, especially for drug testing, raises ethical concerns. Decisions based solely on simulations may have consequences for patients, so ethical considerations around safety, informed consent, and human experimentation must be carefully addressed.
Benefits
- Cost and Time Savings: One of the most significant advantages of biomedical simulation is the potential for substantial cost and time savings. Traditional animal and human trials can be expensive and time-consuming. Simulation allows researchers to explore a wide range of scenarios and test hypotheses rapidly and cost-effectively.
- Safety Improvement: Simulation helps identify potential side effects and safety concerns in the early stages of drug and medical device development. This proactive approach can lead to safer and more effective products, reducing the risk to patients.
- Personalised Medicine: Despite the challenges of variability, biomedical simulation can facilitate personalised medicine by tailoring treatments to individual patient characteristics. By considering genetic, physiological, and lifestyle factors, simulations can help optimize treatment plans.
- Exploration of Inaccessible Scenarios: Simulation enables the exploration of scenarios that are difficult or impossible to replicate in real-life experiments. For example, studying the effects of extreme physical conditions or rare diseases can provide valuable insights that may not be achievable through traditional means.
- Iterative Design and Optimization: Simulation tools, such as Finite Element Analysis (FEA) and Computational Fluid Dynamics (CFD), allow engineers to iteratively design and optimize medical devices, implants, and prosthetics. This iterative process can lead to the development of high-performance products with enhanced safety profiles.
- Non-Invasive Studies: Simulation, particularly in the case of CFD, enables non-invasive studies of physiological processes. This non-invasive approach reduces the risks associated with invasive procedures and provides a better understanding of internal biological phenomena.
The Future of Engineering Simulation in the Medical Sector
The role of engineering simulation in the medical sector is poised for significant growth and innovation in the coming years. As technology continues to advance and our understanding of biological systems deepens, the future of simulation in biomedical engineering holds great promise.
One of the most prominent trends in the future of biomedical simulation is the move toward patient-specific simulations. With the integration of patient data such as genetics, medical history, and real-time physiological data, simulations can be tailored to individuals. This approach has the potential to optimise treatment plans, predict patient responses, and minimise adverse effects.
Artificial Intelligence (AI) and machine learning algorithms will play a pivotal role in enhancing the accuracy and predictive power of biomedical simulations. These technologies can help automate model calibration, improve data integration, and enable simulations to adapt and learn from real-world patient outcomes.
To better understand the complexity of biological systems, future simulations will increasingly employ multiscale modelling techniques. These approaches will bridge the gap between molecular-level interactions and macroscopic physiological responses, providing a more comprehensive view of biological processes.
Real-time simulations will become more common, allowing healthcare professionals to monitor and interact with simulations during surgical procedures or clinical interventions. This real-time feedback can enhance decision-making and improve patient outcomes.
Simulation will continue to reduce the dependency on traditional animal and human trials. Virtual clinical trials, which use large-scale simulations to evaluate the safety and efficacy of drugs or medical devices, will become more reliable and accepted by the healthcare industry and regulatory agencies, accelerating the development and approval process for medical devices and drugs.
Simulations will also play a crucial role in the design and testing of wearable and implantable medical devices. These simulations will help optimize the performance of devices like pacemakers, artificial organs, and neurostimulation implants, ensuring their safety and effectiveness.
The future of biomedical simulation will involve greater collaboration and data sharing among researchers, healthcare institutions, and the pharmaceutical industry. Shared databases of validated models and simulation results will promote transparency and accelerate progress in the field.
As simulations become more integral to medical decision-making, ethical and regulatory frameworks will evolve to address issues related to patient safety, data privacy, and model validation. Ensuring the ethical use of simulation technology will be a critical aspect of its future development.
Conclusion
In conclusion, engineering simulation is enhancing the medical sector, offering a path to faster, safer, and more personalised healthcare solutions. Traditional trials involving animals and humans are costly and time-consuming, and the inherent biological variability poses challenges.
The future of biomedical simulation promises patient-specific models, driven by genetic and physiological data, optimising treatments and minimising adverse effects. Artificial intelligence and multiscale modeling enhance accuracy, while real-time simulations empower surgical decisions. Virtual clinical trials reduce reliance on traditional methods, saving time and resources.
Simulations are also vital for wearable and implantable devices, ensuring their safety and effectiveness. Collaboration and shared databases foster innovation, but ethical and regulatory concerns must be carefully managed.
In silico medicine, simulating complex biological processes on computers, represents the next frontier. Technology advancements and interdisciplinary collaboration hold the key to improving patient outcomes and transforming healthcare. The future of engineering simulation in medicine is marked by innovation, collaboration, and a commitment to delivering safer and more effective treatments.
If this post was helpful to you, consider subscribing to receive the latest blogs and updates! π
And if you would love to see another topic on FEA or CFD, please leave a comment!π
Keep engineering your mind! β€οΈ
Jousef